Our Research Areas
At CMRI, we focus on four key research areas that we believe are crucial to finding cures for serious childhood diseases. These areas are:
Cancer
Cancer occurs when cells from a person's body grow out of control.
Our bodies are made up of trillions of cells, each performing an important function, like lung cells helping us breathe and muscle cells helping us move our arms and legs. These cells wear out and need to be replaced, so copies are made through a process called cell division. Errors can occur during this copying process, errors in the DNA called mutations. DNA contains the blueprint for building a cell and even a whole person, so when there is a mutation it can cause serious problems. One type of problem is cancer.
Most cells in the body have an inbuilt STOP signal that tells them when to stop dividing. For example, skin cells divide approximately 20 times and then they stop. This is important as we need to replace our skin cells; we lose them all the time. Cancer cells, however, can divide 100’s to 1000’s of times, forming tumours that interfere with the normal functioning of the body.
Scientists have found that one of the ways in which cancer cells keep on dividing is for them to maintain the ends of their chromosomes, and these ends are called “telomeres”. Normally these telomeres are gradually lost until they become too short and the cells stop dividing.
Cancer cells counteract the loss of telomeres using:
- Telomerase activity or
- ALT (Alternative Lengthening of Telomeres) mechanism
Telomerase is an enzyme that is active in embryos and foetuses during development. It enables us to develop from a single one-celled embryo into an individual with trillions of cells. Abnormal telomerase activity in cancer cells enables adult cells to continue to divide and form tumours. It is estimated that around 85% of cancer cells have telomerase activity.
ALT is another way by which cancer cells maintain their telomeres. These cells can be identified by ALT-associated bodies seen in the nucleus of tumour cells and stained with a special dye. Approximately 15% of tumour cells use the ALT mechanism to become immortal.
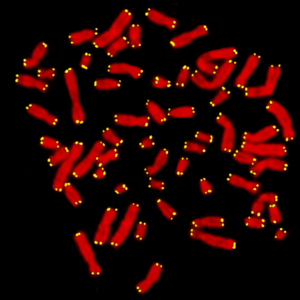
DNA is packaged into chromosomes (stained red) and telomeres are caps at the end (yellow), like the plastic protectors at the end of a shoelace.
These mechanisms of tumour growth and development are being studied by the Cancer Research Unit, Cell Biology Unit, Telomere Length Regulation Group, and Genome Integrity Group at Children’s Medical Research Institute. These groups of researchers are trying different approaches to understand the processes around telomeres, which will be important for the development of new treatments for cancer.
Neurobiology
Nerve cells are the cells of the brain that send electrical signals to each other to control learning, memory and behaviour. They also send signals throughout the body to connect the brain with muscle and other organs.
Nerve cells send electrical signals in one direction: from the dendrite, through the body and down the axon to the nerve terminal at the end of the axon. The point at which the nerve terminal makes contact with other nerves or with muscles is called a synapse. A synapse is the point at which communication between cells occurs, or where instructions for muscle movement are transmitted.
Nerve cells communicate by transmitting an electrical signal from the nerve terminal of the signalling cell to the cell body of the receiving nerve cell or muscle. The electrical signal is transmitted by a chemical called a neurotransmitter. There are many neurotransmitters that perform different roles throughout the body. Glutamate, serotonin, and dopamine are examples of neurotransmitter.
Nerve cells contain neurotransmitter, which is packaged into synaptic vesicles that are stored in the nerve terminal. A process of exocytosis and endocytosis allows a nerve cell to release neurotransmitter, which is detected by the receiving cell.
Every muscle movement or thought is the result of cell communication and requires the release and subsequent reception of neurotransmitter. Memory is also the result of nerve cell communication and relies on how much neurotransmitter is released across how many synapses, and where those synapses are located.
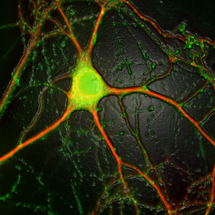
A neuron. The nerve cell body is coloured green and dendrites are coloured red.
Conditions of the brain such as epilepsy, bipolar disorder, depression and possibly Alzheimer’s disease are caused by a malfunction of the cell communication process.
The Cell Signalling Unit at CMRI are dedicated to understanding the exact processes involved in cell communication, in order to find out how we can prevent or treat neurological disorders. They do this by discovering how our genes build a synapse and what the machinery is inside the nerve cells that allow them to work.
They have already made great strides in understanding the function of several proteins involved in cell communication. Recently they discovered that a protein called syndapin plays an important role in nerve cell communication. They are currently investigating the exact mechanism of syndapin function to possibly develop more specific and more effective therapeutic agents to treat epilepsy and possibly some mood disorders.
Embryology
When a sperm fuses with an egg, a single-celled embryo is formed. As the embryo starts to divide, first into 2 cells and then 4, 8, 16 and so on, the cell number doubles each time, at this stage the embryo is simply a ball of identical-looking cells.
At approximately day 3 in the mouse embryo, the cells form into a morula, and at day 4-5 they form a blastocyst.
The blastocyst that is formed after 4-5 days of gestation now contains some clearly defined cell types. The cells in the outer layer will form the cells of the placenta, inside these cells there is a fluid-filled space with a ball of cells called the inner cell mass.
Cells from the inner cell mass are those that will give rise to the whole baby mouse. These cells are special because they have not yet specialised into one specific cell type, such as blood cells, skin cells or brain cells. The process of specialisation is known as differentiation. At Children’s Medical Research Institute (CMRI) we focus on understanding how these cells in the mouse embryo differentiate.
We have over 200 different cell types in our body, and these cells have very different functions. Just consider the role of muscle cells, nerve cells and skin cells. By the time we are born, our body is made up of trillions of cells.
Embryologists are interested in all aspects of the developmental processes before birth. Our research is important as it allows us not only to understand development but also to understand what goes wrong in birth defects.
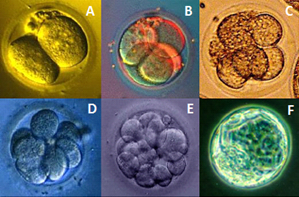
Development of the mouse embryo. (A) two cells, (B) four cells, (C) eight cells, (D) sixteen cells, (E) morula, (F) blastocyst.
Genes carry the information necessary to make proteins in the body. Genes are regulated, or controlled, so that they produce the right amount of protein, in the right part of the body, and at the right time. The regulation of these genes is particularly important in the developing embryo, where genes are “switched on” and “off” in a very precise manner. As an example, genes that produce proteins important for limb development need to be “switched on” during the right stage of development and in the tissues that are destined to form the limbs.
In the Embryology Unit, CMRI scientists have identified a single gene defect that can cause cleft palate. When expression of the gene Pdgfc is “switched off” in mouse embryos, the embryos develop a number of abnormalities, the most significant being a cleft palate, which occurs in nearly 100% of cases.
In a family of several members with cleft palate, the PDGFC gene was found to be non-functional. A mutation was discovered in the promoter of the PDGFC gene. The mutation may cause a problem in “switching on” the gene and thus affects the production of PDGFC protein.
Scientists in the CMRI Embryology Unit are world leaders in fate mapping. They have drawn a map that shows where the cells of the embryo move to during development and what kind of specialised cells they eventually become in the baby and fully grown adult mouse.
An embryo has three layers; the ectoderm, mesoderm and endoderm. Cells of the ectoderm develop into organs such as the brain and spinal cord, cells of the mesoderm develop into tissues such as muscle and bone.
Over the past years, CMRI researchers have made a map of the ectoderm and mesoderm, they are currently looking at the more difficult endoderm layer, a single layer of cells that develop into the gut and other organs such as the pancreas, liver, thyroid and lungs.
Germ cells, the cells that will make up the eggs or the sperm of an individual know their fate from the first few days of life. However, during development they move from their point of origin and hide out near the gut where they wait for the developing ovary or testes to form.
Scientists believe this migration is to enable the germ cells to retain special “stem cell-like” properties, which are important to these unique cells. If they did not migrate, much of the embryo is undergoing extensive remodelling, and differentiation of neighbouring cells could affect the differentiation of these cells.
Two genes (IFITM1 and IFITM3), have been shown by scientists at CMRI to be essential for navigation of the germ cells through the embryo. When both genes are expressed, the cells remain at their point of origin. If IFITM1 is “switched off” and no longer expresses the protein, the cells are repelled from the point of origin and hide next to the gut. When this gene is switched back on the cells can take up residence in the newly formed ovary or testes. By “throwing the switch on and off” for this gene, the cells are guided into the right place.
Genomics and Gene Therapy
Genes are sequences of DNA that code for the proteins in our bodies. Genetic diseases are mostly caused by mutations in a person’s DNA, which affect the quality or amount of a particular protein that is being produced.
To correct a genetic disease, the idea of gene therapy is to use normal, healthy genes as medicine as a way to bypass the genetic cause of the disease. For example, where a patient’s genetic disease is caused by too little of a particular protein, gene therapy may be used to increase the amount of protein in the body.
There are several ways in which genes can be introduced into cells. Examples include:
Microinjection - individually, cell by cell
Gene-gun - shot through the cell membrane of cells
Electroporation - the use of an electrical charge to make the cell membrane “leaky,” enabling the gene/DNA to pass into a cell.
None of these methods are very efficient, so instead we use defective, or “disarmed” viruses as a means to transport genes/DNA into the cells of interest.
One of the viruses used for gene delivery is called adeno-associated virus (AAV). It is a virus that is not known to cause any diseases in humans. In some cases it can stay inside a cell and express a gene for a long time. The AAV virus vector can also genetically modify many different types of cells. Defective AAV virus can efficiently deliver genes to the liver.
The Gene Therapy Research Unit is now preparing for human clinical trials of an AAV-based treatment for inherited liver disease (OTC-deficiency).
AAV may be a safer and more effective alternative to liver transplantation, which is the only current treatment for some children.
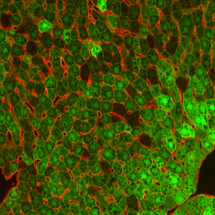
Liver cells infected with AAV and expressing a test gene (GFP) which makes the cells green.
The Gene Therapy Research Unit was the first group in Australia to treat a genetic disease, (SCID-X1, a rare immune deficiency disorder) by gene therapy. X-linked severe combined immunodeficiency (SCID-X1) is a rare immune deficiency disorder and is caused by mutations in a gene called gamma C (the common gamma chain of several interleukin receptors). Affected infants often have an immune system that does not work properly, most lack immune cells called T and natural killer (NK) cells and they have abnormal B cells. As a result, children suffer life-threatening problems fighting infections. Some people call this the “boy-in-the-bubble” disease, because in some countries patients are placed in isolation to stop them from getting infections.